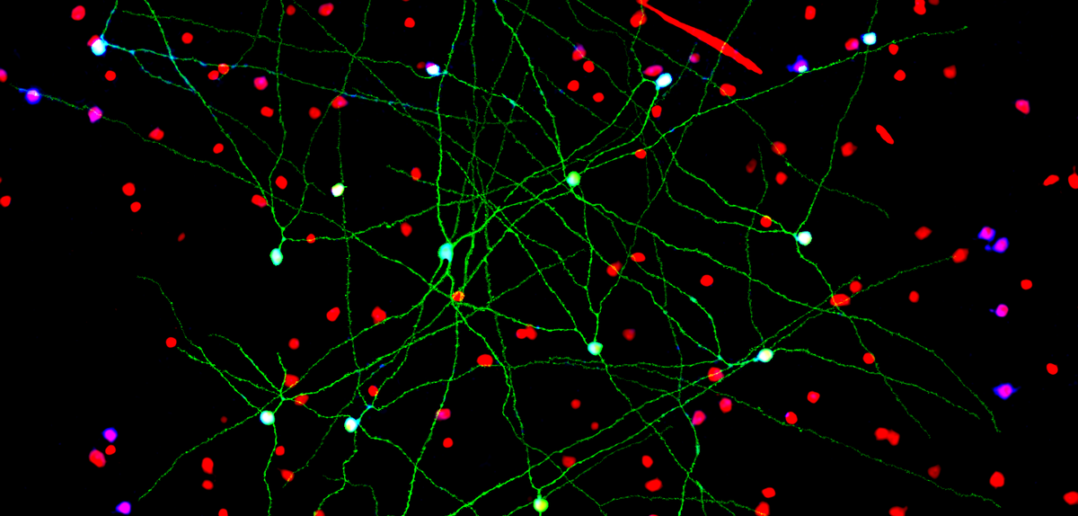
The retina, commonly thought of as a device that takes a “snapshot” of the visual world for further processing by the brain, is now known to be a complex network of cells and synapses that sorts and packages different aspects of the visual scene for transmission to dozens of areas of the brain.
Not only does the retina process images, it also serves as a key part of the central nervous system, helping to regulate everything from daily hormonal oscillations to circadian rhythms.
With advances in technology and genetics, Feinberg investigators are conducting basic science research to understand just how the retina works in concert with the brain.
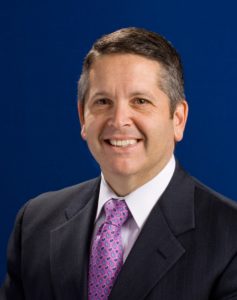
This understanding could ultimately lead to novel treatments for many diseases that lead to blindness, including macular degeneration and diabetic neuropathy.
“The best and most successful treatments of today and in the future are going to depend on a very precise understanding of different cell types, and how those cells communicate with each other and the brain,” said Nicholas Volpe MD, the George W. and Edwina S. Tarry Professor of Ophthalmology and chair of the department. “We’re working to understand exactly what each type of retinal neuron does, what cells it synapses with, how this informs vision processing and ultimately how we might replace or repair damaged cells.”
Mapping Out Retinal Cells
At the heart of much of this area’s research are retinal ganglion cells — the neurons located near the inner surface of the retina. There are about 50 known types of these cells, which transmit information from the retina to more than 50 areas in the brain. Greg Schwartz, PhD, assistant professor of Ophthalmology and Physiology, is working to definitively classify all types of ganglion cells, using the latest technologies and techniques to figure out where they go, and what they do.
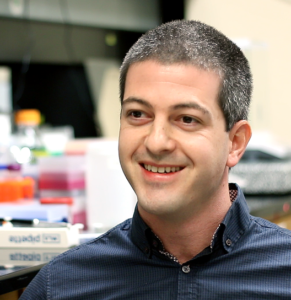
Retinas are particularly interesting to study because they can survive — and continue to function — for many hours outside of the body. That allows Schwartz to use techniques like electrophysiology to study the mouse retina ex vivo. By placing the retina on a slide and showing it movies, he can record the spikes in the ganglion cells that travel down the optic nerve (and ultimately in the brain) to better understand how different visual cues affect different cells. Some ganglion cells are motion-sensitive, while others respond to visual cues like edges or colors.
Schwartz hopes to identify each retinal ganglion cell’s function, genetic signature, and how it is connected to targets in the brain, and after years of research, he estimates that he and other investigators are about 90 percent there. He recently discovered one cell that is likely involved in eye growth, which could ultimately lead to new ways of preventing and treating myopia (nearsightedness).
“We have a real chance of solving the entire retina, of understanding it on a level that we are not close to understanding in the rest of the brain,” he said.
How Retinal Cells Use Proteins Differently
Beyond visual cues, retinas provide the brain with key information that regulates other behaviors, such as our sleep/wake cycles. One subset of these cells, called intrinsically photosensitive retinal ganglion cells, may hold the key to understanding these behaviors.
Tiffany Schmidt, PhD, assistant professor of Neurobiology at the Weinberg College of Arts and Sciences, studies these cells in genetic mouse models. She, too, uses electrophysiology to study how retinal cells respond to movies on a slide versus how the animal with the same genetic disposition behaves in response to the same movies.
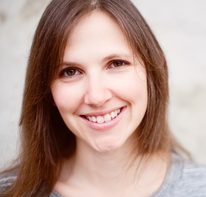
Schmidt recently found that when she removed a protein called melanopsin from retinal cells, the cells could not see contrast as well. This was puzzling because melanopsin was only thought to detect the brightness of environmental light, not image features. She and her group eventually discovered that intrinsically photosensitive retinal ganglion cells all use melanopsin in different ways, depending on the behavior. Some cells use them for contrast, while others use the protein to regulate sleep/wake cycles.
“These cells are all using this protein in unique, specialized ways,” she said. Next, she wants to map how the intrinsically photosensitive retinal ganglion cells are connected to different brain areas to influence these very different behaviors.
“I’m really interested in how we take in information from our environment and how it affects our behavior,” she said. “The visual system is a great model for studying this.”
Following the Signal
But retinal ganglion cells are just one step in a long chain of connections from the eye to the brain.
Steven DeVries, MD, PhD, the David Shoch, MD, PhD, Professor of Ophthalmology, is interested in the first step — how the cone photoreceptor synapse enables the cone to signal to the next neurons in line, the retinal bipolar cells.
To do this, he uses the ground squirrel as a model — since it has a nearly all-cone retina — and records the signals that flow from the cone to the bipolar cell across the synapse. He is also working to grow 3D ground squirrel retinas from stem cells to better understand the proteins expressed in the synapse.
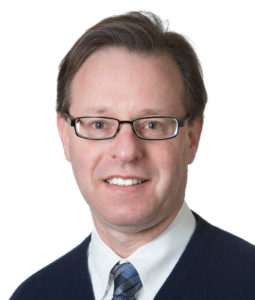
“We want to understand why this synapse has such an unorthodox design and how it functions to support vision,” said DeVries.
DeVries is also collaborating with Yongling Zhu, PhD, assistant professor of Ophthalmology and Physiology, on a project in which retinal ganglion cells are infected with rabies virus. Because the rabies virus spreads rapidly across synapses in the brain, including in the retina, it is a unique tool to understand neuron connections. Rabies allows them to identify the amacrine cells (in the inner retina) and bipolar cells that talk with each type of ganglion cell.
“We want to be able to follow the visual signal as it goes through the retina and into the brain,” he said. “That’s the holy grail — to take a piece of the nervous system and have a complete description of how that works.”
DeVries is equally excited about the clinical possibilities of this knowledge. “Clinicians and scientists are using the same techniques in different ways and developing insights together,” he said.
That sort of basic science to translational science is key in the Department of Ophthalmology, Volpe said.
“We are really of the mindset that when we figure out how these cells communicate with each other, we will be able to make meaningful contributions to reverse vision-affecting diseases,” Volpe said.