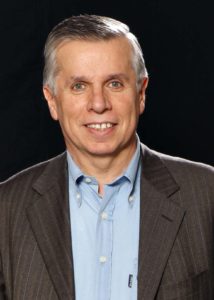
Northwestern Medicine investigators have identified a key interaction in a complex system of checks and balances that keeps cells functioning when they are starved of oxygen, according to a study published in Proceedings of the National Academy of Sciences of the United States of America (PNAS).
About one-third of a cell’s metabolic energy is used to maintain homeostasis using an enzyme called Na,K-ATPase. This enzyme requires a large amount of oxygen, so when a cell’s access to oxygen is restricted, a protein compound called protein kinase C zeta (PKCζ) triggers Na,K-ATPase endocytosis, throttling its metabolic appetite and curbing the cell’s oxygen usage.
Too much throttling, however, can interfere with the basic functioning of the cell, leading to loss of homeostasis and cell death, according to the investigators.
“We are describing a novel mechanism by which Na,K-ATPase is downregulated to allow the cell to survive,” said Jacob Sznajder, MD, Ernest S. Bazley Professor of Asthma and Related Disorders, and senior author of the study.
Investigators identified a chain reaction of transcription factors and ligases that limits PKCζ activation to ensure Na-K-ATPase doesn’t dip below 50 percent of normal levels. Hypoxia-inducible factor 1 (HIF1) upregulates heme-oxidized IRP2 ubiquitin ligase 1L (HOIL-1L), which targets PKCζ for degradation, to preserve cell viability despite the severe oxygen deficit.
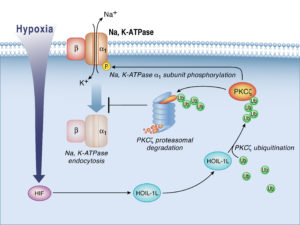
“If you are at a very high altitude, for example, the cell ‘hibernates’ at about half of its metabolic capacity, but it survives,” said Sznajder, who is also a professor of Medicine in the Division of Pulmonary and Critical Care and of Cell and Molecular Biology. “If you didn’t have HIf1 or HOIL-1L, hypoxia would kill the cell.”
This reaction is central to many biological processes, both in healthy and disease states. For example, tumor cells take advantage of this regulatory process to survive, according to co-lead author Laura Dada, PhD, research associate professor of Medicine in the Division of Pulmonary and Critical Care. Natalia Magnani, PhD, postdoctoral research fellow in Sznajder’s lab, was also a co-lead author.
“As the tumor grows, the cells on the inside don’t have any oxygen,” Dada said. “This mechanism allows them to survive.”
While blocking this pathway in cancer cells could theoretically lead to cancer treatments, future therapies exploiting this pathway must be careful to only selectively inhibit it, according to Sznajder.
“When we are just three or four cells, in the womb of our mothers, we are very hypoxic,” he said. “That’s when HIF1 is first activated. It’s that fundamental to early organ development.”
Next, the investigators intend to integrate newer technology and examine cellular perturbations in this process, with a large-scale quantitative approach using single-cell RNA sequencing genomics.
“This current study is an example of a hypothesis-driven approach,” Sznajder said. “We are going to expand the scope of our research and investigate the influence of this process on the whole cell using unbiased ‘omics’ approaches established by our colleagues Drs. Alexander Misharin and Hiam Abdala Valencia.”
This work was supported, in part, by National Institutes of Health grants HL48129, HL-071643, and AG-049665. This work was also supported by the Northwestern University Flow Cytometry Core Facility, supported by National Cancer Institute (NCI) Cancer Center Support grant CA060553. Flow cytometry cell sorting was performed on a BD FACSAria SORP system, purchased through the support of NIH grant 1S10OD011996-01. Imaging work was performed at the Northwestern University Center for Advanced Microscopy, and histology services were provided by the Northwestern University Mouse Histology and Phenotyping Laboratory, both of which are supported by NCI grant CCSG P30 CA060553 awarded to the Robert H. Lurie Comprehensive Cancer Center of Northwestern University.