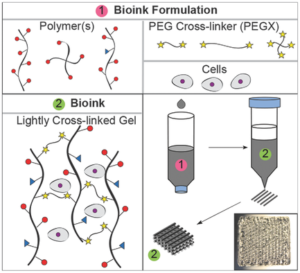
Three-dimensionally printing human tissues and organs is a promising approach to regenerative medicine. But scientists in this field face major challenges, including identifying materials that will maintain their shape after printing and also safely support cell life once inside the human body.
In two recent studies, the lab of Ramille Shah, PhD, assistant professor in Surgery, developed new methods to create viable biomaterials for layer-by-layer 3-D printing, expanding the tools available to scientists to build a vast array of functional artificial structures: those with blood vessels and bile ducts, electrically conductive cardiac muscle and more.
“The main goal of my lab is to spatially organize cells and materials so that they better mimic real organs and tissues,” said Shah, who has a joint appointment at the McCormick School of Engineering in the Department of Materials Science and Engineering.
In the first paper, published in Advanced Materials, she and colleagues came up with a universal process for printing soft gels called hydrogels to form tissues. Cells grow well within this aqueous type of material, which can be made with natural polymers such as gelatin and collagen. However, hydrogels for cell encapsulation are usually physically weak, and can’t maintain a 3-D printed form on their own.
In the past, scientists have increased the polymer concentration of hydrogels or cross-linked polymer chains to thicken or strengthen the material. But these methods can prevent cells from migrating, proliferating and differentiating.
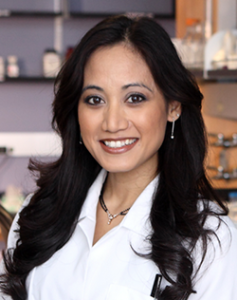
“We need strategies that help the hydrogels hold their shape upon printing, as well as support a compatible environment for cells,” Shah said.
In the study, her group accomplished this task using a commercially available compound called polyethylene glycol (PEG), designed to lightly cross-link both natural and synthetic hydrogels. In this way, the materials can be 3-D printed into well-defined, self-supporting scaffolds with polymer concentrations much lower than ever before. It’s possible to tailor the method – the lab demonstrated 35 different formulations – to accommodate the printing of multiple materials at once, with or without cells, as well as different structural and biological properties depending on the tissue target.
“We’re trying to create a ubiquitous method so that multiple types of biomaterials – for example, ones for vascularization or ones for liver regeneration – can be printed together to create more complex organs and tissue structures,” Shah said.
Graphene Structures for Electrogenic Tissue Engineering
While soft, water-rich hydrogels are ideal for printing materials containing live cells for tissue engineering, solid, particle-based materials can be used to create structural scaffolds that cells are placed onto after printing. In a paper published in ACS Nano, Shah’s group investigated one such biomaterial: graphene.
Composed of nano-sized carbon flakes, graphene is an appealing resource for biomedical applications because it is electrically conductive – a property that can influence cell behavior. In the study, Shah and colleagues printed 3-D structures that were 60 to 70 percent graphene by volume – previously, only about 20 percent graphene had been possible, not enough to preserve the material’s unique properties.
“This is the first time anyone has demonstrated the ability to 3-D print majority-graphene structures at larger scales – multiple centimeters in size – while still maintaining structural integrity and functional properties,” Shah said. “We’re interested in using them as electrically conductive scaffolds to grow cells that respond to electrical signaling, like those in cardiac or nerve tissue.”
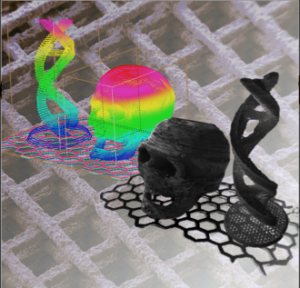
The graphene ink prints as a liquid and dries into a robust, flexible scaffold that can be cut and sutured to tissues in the body or fabricated into patient-specific implants. The scientists also demonstrated that the material is biocompatible and biodegradable in vitro and in vivo.
In one test, they populated a graphene scaffold with stem cells to see if its electrical conductivity influenced cell behavior.
“We showed that these new electrically conducting scaffolds can inherently induce stem cell differentiation into neuron-like cells without the need for additional growth factors or electrical stimuli for nervous tissue regeneration,” Shah said. “It was very surprising.”
Biomedical applications for this work are broad. For example, graphene scaffolds could help regenerate damaged muscles and restore signaling so that movement is more natural, especially in facial defects or abnormalities.
Shah is currently collaborating with faculty from Feinberg’s divisions of Surgery-Organ Transplantation and Surgery-Plastic Surgery and the departments of Orthopaedic Surgery and Obstetrics and Gynecology to explore how these new 3-D printable biomaterials can be used for specific clinical situations.
“We’re just at the beginning,” she said. “We have expanded the biomaterial toolbox for 3-D printing, but now we need to test these materials with different cell types and for different tissue targets.”
The hydrogels work was supported by the National Institutes of Health (grant 1K01DK099454–01) and a National Science Foundation Graduate Research Fellowship. The graphene study was funded by Northwestern University’s International Institute for Nanotechnology (NU# SP0030341), Northwestern University’s McCormick Research Catalyst Award, the Office of Naval Research MURI Program (N00014-11-1-0690) and the Department of Defense through the National Defense Science and Engineering Graduate Fellowship Program.