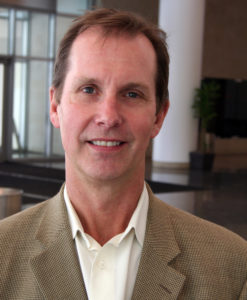
A chance discovery points to a new understanding of how neurons power transmitter release, according to a new study published in Nature Neuroscience.
A team led by D. James Surmeier, PhD, chair and the Nathan Smith Davis Professor of Physiology, uncovered how dopamine-producing neurons use spare electrons — generated by cleaning up excess dopamine — to help their mitochondria generate adenosine triphosphate (ATP), the energy currency of cells.
“It’s a beautiful biological solution to the problem of supplying the energy necessary for transmitter release in these neurons,” Surmeier said.
The most bio-energetically expensive thing neurons do is release chemical transmitters, which requires significant amounts of energy, a strain felt especially by dopamine-producing neurons.
“A normal neuron may have a thousand transmitter sites per axon, but a dopaminergic neuron may have as many as one million sites per axon,” Surmeier said.
Surmeier, along with lead author Steven Graves, PhD, postdoctoral fellow in the Surmeier laboratory, originally wanted to investigate why dopaminergic neurons die in Parkinson’s disease. The prevailing theory is that excess dopamine contributed to this degeneration, as it can be toxic to neurons at high concentrations.
To help clear out excess dopamine, these neurons use monoamine oxidase (MAO), an enzyme anchored to the outer membrane of the mitochondria within neurons. As dopamine waits to be packaged, MAO degrades small amounts and this reaction produces an extra electron.
“We figured this electron wouldn’t just be thrown away,” Surmeier said.
The investigators used optical methods and genetically encoded sensors to track the path of this electron, finding that it was shuttled into the electron transport chain of the mitochondria.
“This helps the mitochondria produce the ATP needed for transmitter release,” Surmeier said.
While this mechanism helps dopamine-producing neurons meet their high energetic demands, there is a potential dark side, according to Surmeier. Many Parkinson’s patients are prescribed a dopamine-boosting drug such as levodopa, which dramatically elevates dopamine production in the remaining neurons.
“This could lead to too much metabolism of dopamine and mitochondrial oxidant stress,” Surmeier said. “These mitochondria are already working hard to power the huge demands of these neurons, and this added stimulus could push them over the edge”
Fortunately, early stage Parkinson’s disease patients are often given a MAO inhibitor that is likely to lower the stress created by dopamine metabolism, according to Surmeier.
“In early stage patients, the combination of levodopa and a MAO inhibitor is very effective and not likely to lead to any acceleration in the disease,” Surmeier said.
Surmeier and his colleagues weren’t specifically looking for the role the mitochondria and MAO plays in Parkinson’s disease — the scientists simply conducted open-ended experiments and explored the results.
“To be honest, we had no plan to study this — sometimes you put your Sherlock Holmes hat on and do some biological detective work,” Surmeier said.
This study was supported by grants from the JPB Foundation, the IDP Foundation, the Michael J. Fox Foundation, NIH grants NS047085, NS076054, DA039253, U01NS103522, U01NS090604 and DPMH107056, a Northwestern Memorial Foundation grant and the Boyd and Elsi Welin Professorship and Tsai Family Fund.