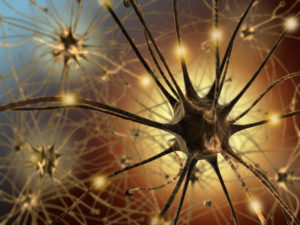
A Northwestern Medicine study has shown how signals from neurons in the motor cortex – the area of the brain that controls voluntary muscle activity – produce precise and consistent movement over time.
The findings, published in the Journal of Neuroscience, have implications for the development of brain machine interfaces, systems that decode brain signals to control external devices like robotic limbs.
“This study presents some of the first evidence from the brain to support a popular theory called optimal feedback control, which explains how the brain controls movement,” said senior author Marc Slutzky, ’02 MD, ’00 PhD, ’06 GME, associate professor of Neurology, Physiology, and Physical Medicine and Rehabilitation. “In particular, this theory seeks to explain how the brain accounts for redundancy in the motor system. There are many different muscles and many millions of neurons that control those muscles, so how does the brain learn to consistently produce the same movements with so many variables?”
To explain optimal feedback control theory, Slutzky used the example of a person repeatedly touching the same button on a tablet while playing a game.
“All that really matters for the task is that your finger touches the right spot. It doesn’t matter what your elbow does as long as you’re accurately touching the button every time,” Dr. Slutzky said. “What happens is the brain learns to control what it needs to in order to accomplish a given task and doesn’t worry about the things that don’t matter. Controlling every single muscle identically every time you reach for the button would require too much energy.”
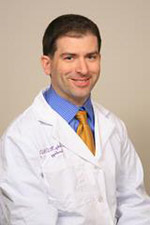
Indeed, using animal models, the research team demonstrated that cortical activity is more stable in task-relevant space (such as the tablet screen) and more variable in task-irrelevant space (like wherever the elbow is). The scientists also discovered that stability in the relevant space lasts over time.
“We showed that brain signals from the motor cortex show extremely stable behavior over very long time periods, up to 3 years,” Dr. Slutzky said. “This is far more stable than anyone had shown before.”
In the study, the scientists recorded two types of electrical signals that neurons in the cortex produce – called action potentials and local field potentials – during a simple task. Though most brain machine interfaces work by deciphering action potentials to communicate to muscles, the scientists found that the other type of signal is actually more stable.
“This suggests we should be looking harder at local field potentials as the main signal source for brain machine interfaces,” said Dr. Slutzky, whose ultimate goal is to design clinically viable brain machine interfaces that help patients paralyzed due to neurological conditions such as stroke, cerebral palsy and ALS. “Being clinically viable means that the machine not only performs well, but also that it’s easy for a patient to learn and that it will last many years.”
Robert Flint, PhD, a postdoctoral fellow in Dr. Slutzky’s lab, and Michael Scheid, a graduate student in the Northwestern University Interdepartmental Neuroscience (NUIN) program, were first authors of the paper. Sara Solla, PhD, professor of Physiology with a joint appointment in Physics and Astronomy at the Weinberg College of Arts and Sciences, was a co-author.
This work was supported by National Institutes of Health grants K08 NS060223, R25 GM79300 and T32 HD057845 and the Defense Advanced Research Projects Agency grant N66001121-4023.